Studying nuclear spin in the nanoworld
A new form of NMR technology gives scientists what they need to examine the structure of minute samples
Making new insights into the nanoworld a reality! An international team working with scientists from the University of Stuttgart and the Max Planck Institute for Solid State Research in Stuttgart have put the conditions in place to examine proteins and other nanostructures with the help of nuclear spin spectroscopy. This is a method that reveals the structure of a material, however, on nanoscopic samples it previously involved enormous technical complexity. As a long-term goal, the Stuttgart-based physicists plan to use their new variant of this technology to observe protein folding and protein complexes. This would bring the sort of new knowledge to the biomedical field that no other method has been capable of offering to date.
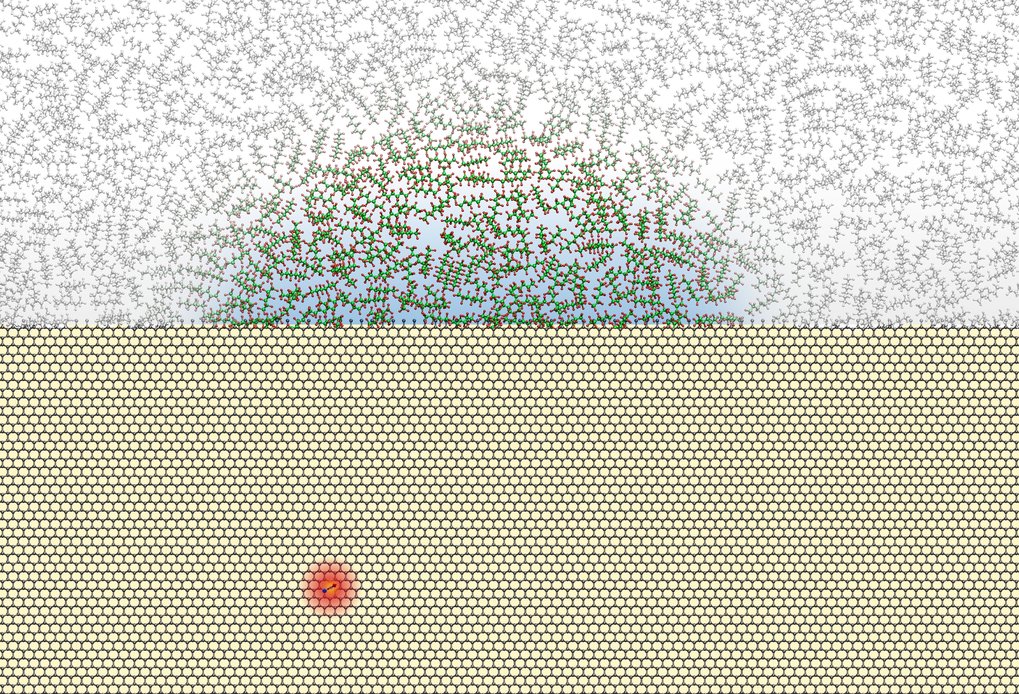
Not many methods offer as detailed a look into the human body as magnetic resonance imaging. Organs and any damage they have suffered can be seen in more detail with this technology than any other. Chemists, too, can learn a great deal about molecular structure with nuclear spin spectroscopy, also known as magnetic resonance or NMR spectroscopy. And now scientists working with Friedemann Reinhard, head of a working group at the University of Stuttgart, and Jörg Wrachtrup, Professor at the University of Stuttgart and Fellow of the Max Planck Institute for Solid State Research, have opened a new window onto the nanoworld with the help of nuclear spin spectroscopy. They have managed to record an NMR signal from a drop of oil with a miniscule volume, the same as would fit into a cube with sides just five nanometres long. At the same time as the group of physicists in Stuttgart, an American team working with IBM researchers is also reporting this foray into the nanodimension of NMR (doi: 10.1126/science.1231540).
Up to now, samples had to be at least three micrometres in size for NMR testing to work. In the medical area, that is not a problem, and chemists, too, can usually find sufficient quantities of an unknown substance in their flask to identify it with NMR spectroscopy. However, things are different in biochemistry. Here, it would often be helpful to examine a single protein – to find out how it is folded, for example. The way in which a protein is folded is decisive for the task it fulfils in the body. While biologists can get this structural information out of the proteins using X-ray crystallography or conventional NMR, both of these methods require countless identical copies of a molecule in order to determine its structure.
A nitrogen atom in a diamond picks up NMR nanosignals
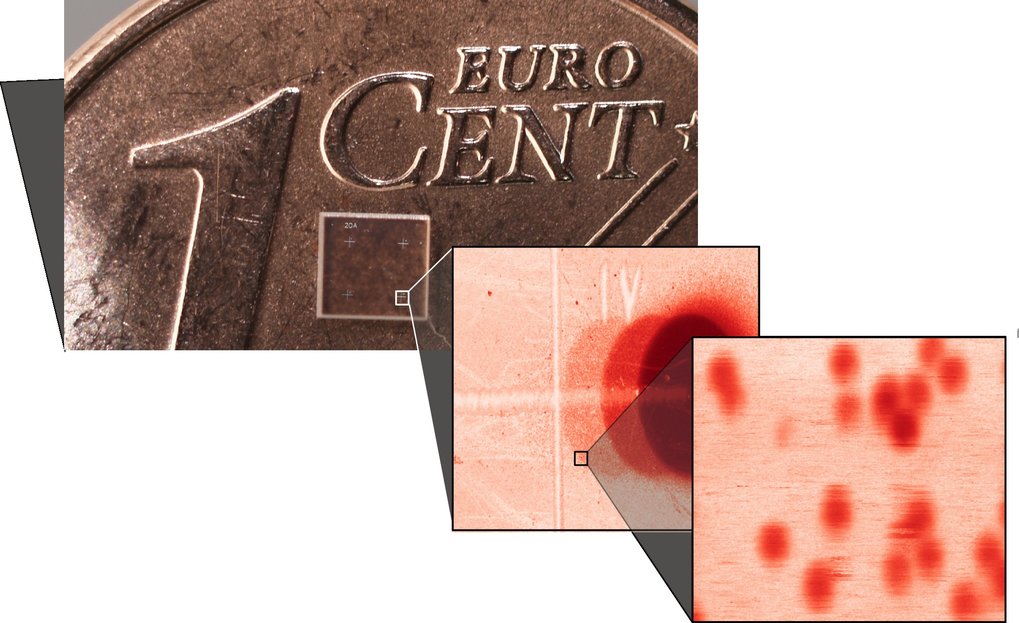
Biologists are interested in how a protein works at least as much, if not more than, the way it is structured. This is why they study complexes of proteins and receptors – insofar as conventional methods allow. NMR spectroscopy of individual complexes would simplify that task greatly. And it is not only biologists who would like to see the nanoworld through the window of NMR – materials scientists have high hopes, too. That's because the tiny structures they work with in nanotechnology are very difficult to penetrate with conventional methods. Above all, what the interior of the structures looks like mostly remains a mystery to them. And NMR spectroscopy could be very helpful here.
On their own, tests on nanoscale samples have not been successful, as there are no detectors for the nuclear spin signals from samples that are as small as a single protein or a protein-receptor complex. "We’ve discovered a detector that can do that, which works at normal ambient temperature and in regular air," says Friedemann Reinhard. "This is a significant step towards the analysis of NMR signals from nanoscopic samples." To date, nanoscale samples could only be examined at temperatures close to absolute zero (minus 273.16 degrees Celsius). Moreover, that could only be done with long testing times and in a very clean vacuum, which requires a great deal of effort to create.
The NMR detector that Reinhard and his fellow scientists used is a diamond with a nitrogen atom built into its carbon lattice just below the surface of the crystal. The physicists call the point at which the foreign atom sits the NV centre: N for nitrogen and V for vacancy, denoting a gap in the carbon lattice right next to the nitrogen atom. It is these NV centres that give some gem-quality diamonds a reddish hue. For the Stuttgart-based physicists, an NV centre is interesting as it detects the nuclear spins of atoms located right above the NV centre on the diamond’s surface.
No strong magnets needed to detect the nuclear spin of nanoscale samples
Nuclear spin is what makes each atom into a tiny bar magnet rotating around an axis, like a sort of lurching spinning top. This axis can be aligned with the help of an external magnetic field, but is otherwise randomly oriented in space. The speed of this spin around the axis depends partly on what else is surrounding the atom. So this rotation speed or frequency tells us something about the structure of a substance.
The human body or a sample for a conventional NMR examination contains a great deal more atoms than there are stars in the universe. And even the tiny drop of oil used by Reinhard's team as the nanoscale sample contains a further 10,000 or so hydrogen atoms. Their nuclear spins are oriented randomly in every possible direction. In a conventional NMR machine, a strong magnet brings order to the chaos. In a nanoscale sample, it is pure chance that helps. Chance is behind the fact that there are almost always up to 100 more nuclear spins going in one direction than in all others. Their little bar magnets then form a slightly larger one – and the sample is magnetically polarised. This larger bar magnet also lurches around an axis, and the speed of its rotations is exactly the same as the speed of the individual atoms' nuclear spins. The rotation speed is not influenced by the orientation of the axis of the bar magnet formed in the course of polarisation.
The rotation speed of the bar magnet formed by random polarisation in a sample on the diamond's surface is detected by a nitrogen atom right underneath the surface on the basis of its own spin. "Unlike with a conventional sample, we do not need strong magnetic fields in order to pick up a signal," says Friedemann Reinhard. This considerably reduces the experimental effort.
A new physical method is needed to sensitise the nuclear spin detector
The scientists in Stuttgart must first sensitise their detector, the NV centre, to the sample's signal because it picks up all kinds of magnetic noise in addition to the signals from the sample. "What we need to do is process the information in the nuclear spins differently to how it is done in conventional NMR spectroscopy," explains Friedemann Reinhard. "So our method is not about merely miniaturising the usual method." At the core of the new physical method is an elaborate sequence of microwave pulses. The scientists vary the length of the pauses between pulses. As soon as the duration of the silence matches the time it takes for the polarisation magnet in the sample to complete half a rotation, the nuclear spin detector in the diamond registers a signal.
Physicists have taught even conventional NMR machines used in medicine and the sciences quite a number of tricks to get the nuclear spins to yield information about the body's interior or the nature of an unknown substance. For the most part, they need to employ ingenious sequences of radiowave pulses and magnetic coils to do so. "There's a lucky coincidence that enables us to detect the magnetic polarisation in the amount of light emitted by the NV centre. It means we can read it out with a microscope. What’s more, we can manipulate it with microwaves and laser light," says Friedemann Reinhard. And it's thanks to this freak of nature that it might prove possible to realise an NMR machine for nanoscale samples with less technological effort than conventional nuclear spin devices.
Nitrogen atoms in diamonds are like the processor in a quantum computer
Nevertheless, it will be some time yet until a nuclear spin examination of a nanosample can tell us as much as that about the body or the content of a chemical flask. "What we have done in the first instance is demonstrate that NMR signals can be detected in nanoscale samples," says Friedemann Reinhard. "Now we need to work on extracting from these signals information that can tell us about the structure of a sample."
However, the physicists are interested in an application very different to the analysis of bodies or materials: "The protocol we use to sensitise the NV centre can be viewed as a quantum algorithm." And this is one of the directions in which the physicists in Stuttgart will continue to explore their system. For instance, single nitrogen atoms in diamonds may, in future, be more than just detectors for NMR signals – they may serve as processors for quantum computers, which are expected to one day be able to process information much faster than conventional computers.
PH