Understanding the Role of Hydrogen and Oxygen in Electronic Phase Changes of Nickelates
Hydrogen is the most abundant element in the universe. This makes it attractive for use in sustainable technologies, such as energy storage and fuel cells, but also in novel electronic components. In contact with transition metal-oxygen compounds, it can reversibly change electrical resistance or magnetism, thereby creating functionality. However, three fundamental questions always arise: how much hydrogen is in the material, in what form is it incorporated and how stable is the compound?
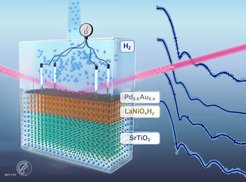
Scientists at the MPI-FKF investigated these questions for the transition metal oxide LaNiO3 and recently published their results in the paper "Understanding the Role of Hydrogen and Oxygen in the Electronic Phase Changes of Nickelates" in Advanced Functional Materials. Using a combination of different measurement techniques, the scientists were able to correlate the exact composition of oxygen and hydrogen in the material with its resistance changes, a behaviour that could be relevant to many compounds.
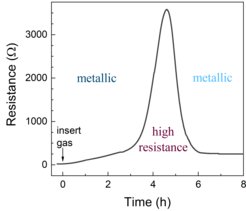
The compound LaNiO3 is a good conductor, but when exposed to hydrogen, the electrical resistance increases significantly. If a thin film of LaNiO3 with an even thinner catalyst layer is produced and sufficient hydrogen can be uniformly incorporated, the electrical conductivity becomes as good as in the initial phase (Fig. 1). In principle, such a material system could be used as a hydrogen sensor, a switch or for more exotic components, such as electronics that replicate the human brain, since the thin catalyst layer used in this study allows the reaction to take place even at room temperature and in atmospheres with small amounts of hydrogen gas. Importantly, the study shows that the incorporation of hydrogen into LaNiO3 is not independent of changes in oxygen content. As hydrogen is incorporated, some oxygen is removed from the material, and the interaction of these processes leads to the observed change in electrical resistance over time (Fig. 1).
A mutual influence of hydrogen and oxygen content probably also occurs in other functional transition metal oxides, and the methodological approach of this work can be applied to a variety of samples.
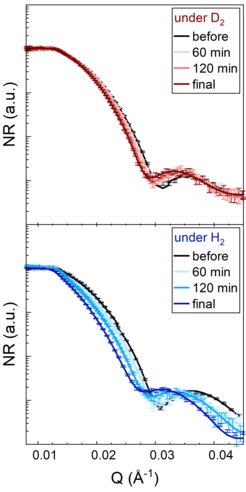
So how exactly do we determine the amount of hydrogen and oxygen in LaNiO3? The most common method for determining the structure of compounds is x-ray diffraction, but light elements with low electron density, such as hydrogen and oxygen, are almost invisible to x-rays. It is therefore difficult to quantify hydrogen, and many methods require large amounts of sample or lead to destruction of the material, so they cannot be carried out during the reaction. Neutron scattering is an exception, because neutrons scatter particularly strongly from hydrogen and less strongly from its isotope deuterium, even though both behave in the same way in the chemical reaction. Figure 2 shows neutron reflectivity curves of the LaNiO3 film in hydrogen and deuterium atmospheres at different times. The differences are clearly visible, and since the same amount of hydrogen and deuterium is incorporated at the end, the hydrogen content can be quantified. With a little more calculation, this is also possible for the intermediate stages of the reaction. At the same time, the data allow the oxygen content to be quantified and show that the hydrogen incorporation is accompanied by an oxygen loss. This is an interesting observation as the oxygen loss alone also changes the resistance of LaNiO3. When the hydrogen atmosphere is removed, the hydrogen leaves the material, leaving an oxygen-reduced compound with semiconducting properties. If this compound is heated in air, the original state of the material can be restored by adding oxygen. The cycle is complete, the sample becomes highly conductive again and the process can begin again.
Many functional materials contain hydrogen and oxygen with high mobility under ambient conditions. For this reason, in-situ studies such as those presented here are important to gain a better understanding of the reactions that take place and, ultimately, to be able to use them in a controlled manner.